Introduction and history of meteorology and climatology
Chapter – 1
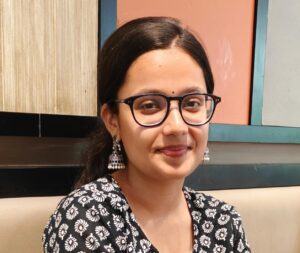
THE ATMOSPHERE
- The atmosphere, essential for terrestrial life, is only 1% of the Earth’s radius in thickness.
- It reached its current form and composition at least 400 million years ago, concurrent with significant land vegetation development.
- The atmosphere rests on the ocean surface, which currently covers 70% of the Earth’s surface.
- Air and water share similar physical properties but differ in compressibility: air is compressible, water is incompressible.
- The study of the atmosphere has a long history involving both observations and theoretical developments.
- Scientific measurements of the atmosphere became possible with the invention of appropriate instruments, which had a long and complex evolution.
- Galileo invented a thermometer in the early 1600s, but accurate liquid-in-glass thermometers with calibrated scales were developed later by Fahrenheit (early 1700s) and Celsius (1740s).
- In 1643, Torricelli demonstrated atmospheric pressure using a column of water or mercury.
- Pascal used Torricelli’s barometer to show that atmospheric pressure decreases with altitude by conducting experiments on the Puy de Dôme in France.
- Boyle, in 1660, demonstrated the compressibility of air and proposed Boyle’s law, stating that volume is inversely proportional to pressure.
- Charles showed in 1802 that air volume is directly proportional to temperature.
- By the end of the nineteenth century, the four major constituents of the dry atmosphere were identified: nitrogen (78.08%), oxygen (20.98%), argon (0.93%), and carbon dioxide (0.035%).
- In the twentieth century, it was noted that CO2 levels had increased significantly, rising by 25% since 1800 and 7% since 1950, mainly due to respiration and industrial activities.
- The hair hygrograph, designed to measure relative humidity, was invented by de Saussure in 1780.
- Rainfall records in England date back to the late seventeenth century, with earlier measurements described in India (4th century BC), Palestine (around AD 100), and Korea (1440s).
- Luke Howard devised a cloud classification scheme in 1803, which was fully developed and implemented in observational practice by the 1920s.
- Establishing networks of observing stations with standardized procedures and rapid data exchange (via telegraph) was crucial and occurred in Europe and North America in the 1850s to 1860s.
- Water’s greater density compared to air gives it a higher specific heat; more heat is required to raise the temperature of a cubic meter of water by 1°C than that of air by the same amount.
- The top 10–15 cm of ocean waters contain as much heat as the entire atmosphere.
- During evaporation, heat energy is translated into the kinetic energy of water vapor molecules (latent heat), as demonstrated by Black in 1760.
- Condensation in a cloud or as fog releases kinetic energy, returning as heat energy.
- The amount of water stored in water vapor depends on air temperature, leading to significant latent heat release when warm moist tropical air condenses, increasing the instability of tropical air masses.
- Convection involves heated air expanding, decreasing in density, and rising, potentially causing precipitation, while cooling air contracts, increases in density, and subsides.
- The combined use of the barometer and thermometer enabled the investigation of the atmosphere’s vertical structure.
- A low-level temperature inversion was discovered in 1856 at about 1 km on a mountain in Tenerife, where temperature ceased to decrease with height.
- The Trade Wind Inversion, found over eastern subtropical oceans, involves subsiding dry high-pressure air overlying cool moist maritime air near the ocean surface, inhibiting vertical air movements and limiting atmospheric activity.
- In the 1920s, the Trade Wind Inversion was shown to vary in elevation between 500 m and 2 km across different parts of the Atlantic Ocean within the belt 30°N to 30°S.
- Around 1900, balloon flights revealed a continuous and widespread temperature inversion at about 10 km at the equator and 8 km at high latitudes, marking the tropopause.
- The tropopause marks the top of the troposphere, within which most weather systems form and decay.
- By 1930, balloons equipped with instruments (radiosondes) measuring pressure, temperature, and humidity, and reporting them back to earth via radio, were routinely used to investigate the atmosphere.
SOLAR ENERGY
- Exchanges of potential (thermal) and kinetic energy occur on a large scale in the atmosphere as potential energy gradients produce thermally forced motion.
- Differential heating of low and high latitudes drives both atmospheric and oceanic circulations.
- About half of the solar energy entering the atmosphere as short-wave radiation (insolation) reaches the Earth’s surface.
- The Earth’s surface, whether land or ocean, re-radiates this heat as long-wave thermal radiation.
- Increased heating of tropical regions compared to higher latitudes was long observed.
- In 1830, Schmidt calculated heat gains and losses for each latitude by incoming solar radiation and outgoing re-radiation from the Earth.
- Schmidt’s calculations showed an excess of incoming energy over outgoing energy equatorward of latitudes 35°, and a deficit poleward of those latitudes.
- The equator–pole thermal gradients result in a poleward flow (flux) of energy, interchangeably thermal and kinetic, peaking between latitudes 30° and 40°.
- This energy flux powers the global-scale movements of the atmosphere and oceanic waters.
- The amount of solar energy received and re-radiated from the Earth’s surface can be computed theoretically by mathematicians and astronomers.
- Following Schmidt, calculations were made by Meech (1857), Wiener (1877), and Angot (1883) to determine extraterrestrial insolation at the outer limits of the atmosphere at all latitudes.
- Milankovitch (1920, 1930) provided theoretical calculations of past insolation, contributing to the understanding of astronomic controls of climate.
- Simpson (1928-1929) calculated the insolation balance over the Earth’s surface.
- Accurate determination of solar radiation received by the Earth was only achieved with satellite measurements in the 1990s.
GLOBAL CIRCULATION
- The first attempt to explain global atmospheric circulation was based on a simple convectional concept.
- In 1686, Halley associated the easterly trade winds with low-level convergence on the equatorial belt of greatest heating (the thermal equator).
- Halley proposed that these low-level flows were compensated by return flows aloft, with air cooling and subsiding poleward of these convectional regions to feed the northeasterly and southeasterly trades at the surface.
- This simple mechanism had two significant problems: explaining the high-pressure in the subtropics and the belts of dominantly westerly winds poleward of this high-pressure zone.
- In 1883, Teisserenc de Bort produced the first global mean sea-level map showing the main zones of anticyclones (high pressure) and cyclones (low pressure).
- Halley’s climatic contribution also included his thermal convectional theory for the origin of the Asiatic monsoon, based on the differential thermal behavior of land and sea.
- Halley suggested that land heats and cools faster than the ocean, causing lower continental pressures in summer and higher pressures in winter, leading to seasonal wind reversals.
- The role of seasonal movements of the thermal equator in monsoon systems was recognized much later.
- Hadley addressed some of Halley’s theory’s difficulties in 1735, focusing on the deflection of winds on a rotating globe (right in the northern hemisphere, left in the southern hemisphere).
- Hadley proposed a thermal circulatory mechanism but was puzzled by the existence of westerlies.
- Coriolis provided a mathematical analysis of moving bodies on a rotating earth in 1831.
- In 1856, Ferrel developed the first three-cell model of hemispherical atmospheric circulation, suggesting a mechanism for high pressure production in the subtropics (around 35°N and S latitude).
- Ferrel suggested that cold upper air subsides in the subtropics, and the deflective force of terrestrial rotation on upper air moving poleward above the Trade Wind Belt causes a build-up of air and pressure in the subtropics.
- Equatorward of these subtropical highs, thermally direct Hadley cells dominate the Trade Wind Belt.
- Poleward of the subtropical highs, air flows towards higher latitudes at the surface, increasingly deflected with latitude, forming the westerly winds in both hemispheres.
- In the northern hemisphere, the northern margin of the westerlies, undercut by polar air moving equatorward, was termed the Polar Front by Bergeron in 1922.
- Ferrel’s three-cell model consisted of two thermally direct Hadley cells (warm air rises, cool air sinks), separated by a weak, indirect Ferrel cell in mid-latitudes.
- Buys-Ballot demonstrated the relationship between pressure distribution and wind speed and direction in 1860.
CLIMATOLOGY
- During the nineteenth century, a large body of global climatic data was assembled, enabling useful regional generalizations.
- In 1817, Alexander von Humboldt produced a valuable treatise on global temperatures, including a map of mean annual isotherms for the northern hemisphere.
- In 1848, Dove published the first world maps of monthly mean temperature.
- Berghaus produced an early world map of precipitation in 1845.
- In 1882, Loomis created the first world map of precipitation using mean annual isohyets.
- In 1886, de Bort published the first world maps of annual and monthly cloudiness.
- These generalizations led to attempts at regional climate classification in the later decades of the century.
- In the 1870s, Wladimir Koeppen, a biologist trained in St. Petersburg, began producing climate maps based on plant geography, as did de Candolle (1875) and Drude (1887).
- Hann’s massive three-volume “Handbook of Climatology” appeared in 1883, remaining a standard until Koeppen and Geiger’s five-volume work of the same title replaced it in 1930–40.
- After World War I, Koeppen produced the first detailed classification of world climates based on terrestrial vegetation cover in 1918.
- Thornthwaite’s classification of climates (1931–33) employed evaporation and precipitation amounts, and in 1948 he introduced the concept of potential evapotranspiration.
- The inter-war period saw the emergence of climatic ideas not realized until the 1950s, including weather type frequencies (Federov, 1921), temperature and rainfall variability concepts (Gorczynski, 1942, 1945), and microclimatology (Geiger, 1927).
- Despite challenges in obtaining detailed ocean measurements, significant climatic research on pressure and wind distributions occurred in the late nineteenth century.
- In 1868, Buchan produced the first world maps of monthly mean pressure.
- Coffin composed the first world wind charts for land and sea areas in 1876.
- In 1883, Teisserenc de Bort produced the first mean global pressure maps, showing cyclonic and anticyclonic ‘centres of action’ underpinning general circulation.
- In 1887, de Bort began creating maps of upper-air pressure distributions.
- His 1889 world map of January mean pressures in the lowest 4 km of the atmosphere effectively depicted the great belt of the westerlies between 30° and 50° north latitudes.
MID-LATITUDE DISTURBANCES
- Theoretical ideas about the atmosphere and weather systems evolved in part due to nineteenth-century mariners’ needs for information about winds and storms, especially predictions of future behavior.
- At low levels in the westerly belt (approximately 40° to 70° latitude), there is a complex pattern of moving high and low pressure systems, while between 6000 m and 20,000 m, there is a coherent westerly airflow.
- Dove (1827 and 1828) and Fitz Roy (1863) supported the ‘opposing current’ theory of cyclone (depression) formation, suggesting energy for the systems was produced by converging airflow.
- Espy (1841) clarified a convection theory of energy production in cyclones, identifying the release of latent heat as the main source.
- In 1861, Jinman proposed that storms develop where opposing air currents form lines of confluence (later termed ‘fronts’).
- Ley (1878) provided a three-dimensional picture of a low-pressure system with a cold air wedge behind a sharp temperature discontinuity cutting into warmer air.
- Abercromby (1883) described storm systems in terms of closed isobars with typical associated weather types.
- By this time, a picture emerged of mid-latitude storms being generated by the mixing of warm tropical and cool polar air due to latitudinal gradients created by incoming solar radiation and outgoing terrestrial radiation.
- Towards the end of the nineteenth century, two important European research groups were studying storm formation: the Vienna group under Margules (including Exner and Schmidt) and the Swedish group led by Vilhelm Bjerknes.
- Margules (1901) showed that the potential energy of a typical depression is less than 10% of the kinetic energy of its constituent winds.
- V. Bjerknes’ group in Stockholm focused on frontal development, with important contributions from 1917 to 1929 after J. Bjerknes moved to Bergen and collaborated with Bergeron.
- In 1918, the warm front was identified; the occlusion process was described in 1919, and the full Polar Front Theory of cyclone development was presented in 1922 by J. Bjerknes and Solberg.
- After about 1930, meteorological research increasingly focused on the importance of mid- and upper-tropospheric influences for global weather phenomena.
- This research was led by Sir Napier Shaw in Britain and by Rossby, with Namias and others, in the USA.
- The airflow in the 3-10 km high layer of the polar vortex of the northern hemisphere westerlies forms large-scale horizontal (Rossby) waves due to terrestrial rotation.
- The influence of Rossby waves was simulated by rotation ‘dish pan’ experiments in the 1940s and 1950s.
- The number and amplitude of Rossby waves depend on the hemispheric energy gradient, or ‘index’.
- During high index periods, especially in winter, there may be as few as three Rossby waves of small amplitude, resulting in a strong zonal (west to east) flow.
- A lower hemispheric energy gradient (low index) is characterized by four to six Rossby waves of larger amplitude.
- Observations in the 1920s and 1930s, and particularly by aircraft observations in the Second World War, revealed that the upper westerlies possess narrow high-velocity threads termed ‘jet streams’ by Seilkopf in 1939.
- The higher and more important jet streams lie along the Rossby waves and clearly affect surface weather by guiding low-pressure systems.
- Subsiding air beneath the jet streams strengthens the subtropical high-pressure cells.
TROPICAL WEATHER
- Success in modeling the life cycle of the mid-latitude frontal depression led to attempts to apply it to tropical atmospheric conditions (30°N to 30°S) in the pre-Second World War period.
- This attempt largely failed due to the lack of frontal temperature discontinuities between air masses and the absence of a strong Coriolis effect and Rossby-like waves.
- Tropical airmass discontinuities are based on moisture differences, and tropical weather results mainly from strong convectional features such as heat lows, tropical cyclones, and the intertropical convergence zone (ITCZ).
- The instability of tropical airmasses means that even mild convergence in the trade winds gives rise to atmospheric waves traveling westward with characteristic weather patterns.
- Above the Pacific and Atlantic Oceans, the ITCZ is quasi-stationary with a latitudinal displacement annually of 5° or less.
- Elsewhere, the ITCZ varies between latitudes 17°S and 8°N in January and between 2°N and 27°N in July, during the southern and northern summer monsoon seasons, respectively.
- The seasonal movement of the ITCZ and other convective influences make the south and east Asian monsoon the most significant seasonal global weather phenomenon.
- Satellite observations after about 1960 assisted investigations of weather conditions over the tropical oceans.
- Observations of waves in the tropical easterlies began in the Caribbean during the mid-1940s, but the structure of mesoscale cloud clusters and associated storms was recognized only in the 1970s.
- Satellite observations also proved valuable in detecting the generation of hurricanes over the tropical oceans.
- In the late 1940s and subsequently, significant work was conducted on the relations between the south Asian monsoon mechanism, the westerly subtropical jet stream, the Himalayan mountain barrier, and the displacement of the ITCZ.
- The failure of the Indian summer monsoon in 1877 led researchers like Blanford (1860), Todd (1888), and others to seek correlations between Indian monsoon rainfall and other climatic phenomena such as Himalayan snowfall and the strength of the southern Indian Ocean high-pressure center.
- These correlations were intensively studied by Sir Gilbert Walker and his co-workers in India between about 1909 and the late 1930s.
- In 1924, Walker identified the ‘Southern Oscillation’—an east–west seesaw of atmospheric pressure and resulting rainfall (negative correlation) between Indonesia and the eastern Pacific.
- Other climatic oscillations were identified in the North Atlantic (Azores vs. Iceland) and the North Pacific (Alaska vs. Hawaii).
- In the phase of the Southern Oscillation with high pressure over the eastern Pacific, westward flowing central Pacific surface water, with consequent upwelling of cold, plankton-rich water off the coast of South America, is associated with ascending air and heavy summer rains over Indonesia.
- Periodic weakening and breakup of the eastern Pacific high-pressure cell lead to subsiding air and drought over India and Indonesia and the removal of the cold coastal upwelling off the South American coast, causing the presence of warm coastal water termed ‘El Niño.’
- The cause of the east Pacific pressure change leading to El Niño is not yet fully understood.
- Interest in the Southern Oscillation waned during the 1940s to mid-1960s.
- Research revived due to the work of Berlage (1957), an increase in Indian droughts from 1965 to 1990, and the strong El Niño in 1972 which caused immense economic hardship.
- A thorough study of ‘teleconnections’ (correlations between climatic conditions in widely separated regions of the earth) pointed out by Walker, became a feature of the renewed research interest.
PALAEOCLIMATES
- Prior to the mid-twentieth century, thirty years of record was generally regarded as sufficient to define a given climate.
- By the 1960s, the idea of a static climate was recognized as untenable.
- New approaches to palaeoclimatology were developed in the 1960s to 1970s.
- The astronomical theory of climatic changes during the Pleistocene proposed by Croll (1867) and developed mathematically by Milankovitch seemed to conflict with evidence for dated climate changes.
- In 1976, Hays, Imbrie, and Shackleton recalculated Milankovitch’s chronology using powerful new statistical techniques.
- They showed that Milankovitch’s chronology correlated well with past temperature records.
- This correlation was especially notable for ocean palaeotemperatures derived from isotopic (O18/O16) ratios in marine organisms.”
THE GLOBAL CLIMATE SYSTEM
- The recognition of the existence of the global climate system was a crucial outcome of work in the second half of the twentieth century.
- The climate system involves not just atmospheric elements but five major subsystems:
- The atmosphere (the most unstable and rapidly changing).
- The ocean (very sluggish in terms of its thermal inertia, important in regulating atmospheric variations).
- The snow and ice cover (the cryosphere).
- The land surface with its vegetation cover (the lithosphere and biosphere).
- Physical, chemical, and biological processes take place within and among these complex subsystems.
- The most important interaction occurs between the highly dynamic atmosphere and the oceans.
- The atmosphere inputs solar energy into the system.
- The oceans store and transport large amounts of energy (especially thermal), acting as a regulator to more rapid atmospheric changes.
- The terrestrial biosphere influences incoming radiation and outgoing re-radiation.
- Human transformations of land cover, such as deforestation and agriculture, affect atmospheric composition via greenhouse gases.
- Marine biota in the oceans play a major role in the dissolution and storage of CO2.
- All subsystems are linked by fluxes of mass, heat, and momentum, forming a very complex whole.
- The driving mechanisms of climate change, referred to as ‘climate forcing,’ can be divided into:
- External forcing (astronomical effects on incoming short-wave solar radiation).
- Internal forcing (alterations in atmospheric composition affecting outgoing long-wave radiation).
- Direct solar radiation measurements have been made via satellites since about 1980.
- The correlation between small changes in solar radiation and the thermal economy of the global climate system remains unclear.
- Increases in greenhouse gas content in the atmosphere due to intensified human activities have been significant.
- These gases include carbon dioxide, methane, nitrous oxide, and ozone.
- The increased greenhouse gases have raised atmospheric temperatures by trapping more terrestrial long-wave radiation.
- The imbalance between incoming solar radiation and outgoing terrestrial radiation is termed ‘forcing.’
- Positive forcing implies a heating up of the system.
- Adjustments to this imbalance occur in months in surface and tropospheric subsystems but take centuries or longer in the oceans.
- Water vapour is the major greenhouse gas, and the effects of changes in water vapour and cloudiness are still poorly understood.
- The natural variability of the global climate system depends on:
- Variations in external solar forcing.
- Features of the system itself, such as feedback and non-linear behavior.
- Major feedbacks in the climate system include:
- The role of snow and ice reflecting incoming solar radiation.
- Atmospheric water vapor absorbing terrestrial re-radiation.
- Both feedbacks are positive, amplifying warming.
- Example: Earth warms → atmospheric water vapor increases → greenhouse effect increases → further warming.
- Example: Higher temperatures reduce snow and ice cover → land or ocean absorbs more radiation → further warming.
- Clouds have a complex role:
- Reflect solar (short-wave) radiation.
- Trap terrestrial outgoing radiation.
- Negative feedback, which dampens changes, is less significant in the climate system.
- This partly explains the recent tendency towards global warming.
- Changes in atmospheric composition due to human actions contribute to climate variability:
- Increases in greenhouse gases lead to global temperature increases.
- Increases in particulate matter (carbon, mineral dust, aerosols) affect climate.
- Particulates, including volcanic aerosols, have a complex influence:
- Some cause atmospheric heating.
- Others cause cooling.
- Numerical models of the atmosphere and climate systems, developed since the 1960s, aid in understanding the global climate system:
- These models handle non-linear processes (which do not have simple proportional cause-effect relationships) and operate on various timescales.
- The aim of this book is to provide a non-technical account of how the atmosphere works:
- Helps understand weather phenomena and global climates.
- The eighth edition of the book has expanded to include many recent advances in climate science.