The Earth as a Rotating Planet
Chapter – 1
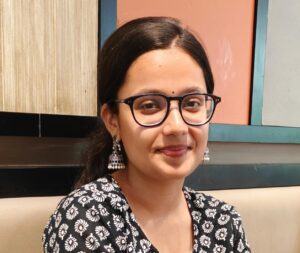
Table of Contents
The Shape of the Earth
- Earth’s shape is nearly spherical.
- Astronaut and satellite photos show Earth as a rotating ball in space.
- Historically, many believed the Earth was flat, especially early sailors.
- Ancient sailors, seeing a flat ocean and circular horizon, feared falling off the edge.
- The Sun’s movement across clouds after setting indicates a spherical Earth.
- Earth is not perfectly spherical; it is an oblate ellipsoid.
- Equatorial diameter: 12,756 km (7926 mi); Polar diameter: 12,714 km (7900 mi).
- Earth’s rotation causes a slight equatorial bulge and polar flattening.
- Earth’s shape is closer to an oblate ellipsoid due to the rotation-induced bulge.
- The geoid is an even more accurate representation of Earth’s shape.
- The geoid is based on gravitational pull variations and is used in mapmaking and navigation.
The Earth’s Rotation
- Earth spins slowly on its axis, an imaginary line through its center and poles.
- Rotation defines a solar day, divided into 24 hours.
- North and South Poles are the points where the axis of rotation emerges.
- Earth’s rotation direction is shown in Figure 1.3.
- Rotation serves as a reference for the geographic grid of latitude and longitude.
- Provides the day as a measure of time, divided into hours, minutes, and seconds.
- Important effects on physical and life processes on Earth.
ENVIRONMENTAL EFFECTS OF THE EARTH’S ROTATION
- Daily rhythms of the Sun govern all forms of life on Earth’s surface.
- Green plants store solar energy during the day and consume it at night.
- Animals have activity patterns based on the day-night cycle.
- The day-night cycle sets the daily air temperature cycle.
- Earth’s rotation affects the directions of large motions in the atmosphere and oceans, causing paths to curve (Coriolis effect).
- Earth’s rotation and the Moon’s gravitational pull cause ocean tides.
- Tides influence many plants, animals, and human activities in coastal areas.
- The Sun also influences tides, as discussed in Chapter 16.
The Geographic Grid
PARALLELS AND MERIDIANS
- Impossible to lay a flat sheet over a sphere without creasing, folding, or cutting.
- Earth’s curved surface poses challenges for mapmakers.
- Cannot divide Earth into a rectangular grid; instead, use the geographic grid.
- Geographic grid consists of imaginary circles: parallels and meridians (Figure 1.4).
- Parallels of latitude are created by slicing the globe like onion rings.
- The Equator is the Earth’s longest parallel and serves as a reference line.
- Meridians of longitude are created by slicing the Earth through its axis like lemon wedges.
- Meridians connect the poles; parallels and meridians define geographic directions.
- Infinite number of parallels and meridians can be drawn, defining unique positions on Earth.
- Great circles are formed when a plane intersects Earth’s center, bisecting the globe.
- Small circles are formed when a plane intersects Earth’s surface but not its center.
- Meridians are halves of great circles; all parallels except the Equator are small circles.
- Great circles can pass through any two points on the globe, representing the shortest distance.
LATITUDE AND LONGITUDE
- Parallels and meridians are labeled by latitude and longitude (Figure 1.6).
- Equator divides the globe into the northern and southern hemispheres.
- Parallels identified by angular distance from the Equator (0° to 90°); labeled N or S.
- Meridians identified by longitude; measured east or west from the prime meridian.
- Prime meridian (0° longitude) passes through Greenwich, England (Figure 1.7).
- Longitude ranges from 0° to 180°, east or west (E or W).
- Latitude and longitude pinpoint locations on the geographic grid (Figure 1.8).
- Fractions of latitude/longitude described in minutes and seconds; 1 minute = 1/60 of a degree, 1 second = 1/60 of a minute.
- Example: 41° 27′ 41″ N = 41.4614° N in decimal notation.
- Degrees of latitude measure north-south distance; 1 degree ≈ 111 km (69 mi).
- Degree of longitude distance decreases with latitude; at 60°, 1 degree ≈ 55.5 km (34.5 mi).
- Elevation (height or altitude) complements latitude and longitude; usually measured from sea level.
- Elevation used in three-dimensional mapping to create realistic terrain maps.
Map Projections
- Cartographers have long grappled with displaying the Earth’s curved surface on flat maps (Figure 1.9).
- Early maps were limited by knowledge and often reflected political or religious views rather than geographic reality.
- Ancient Greek maps (6th century BC) showed the world as an island with Greece at the center.
- Medieval maps (14th century) placed Jerusalem at the center.
- By the 15th century, explorers like Columbus and Magellan expanded the known world.
- Explorers brought mapmakers to record new lands, making navigation charts highly valued.
- Representing the Earth’s curvature on a flat page became a significant challenge.
- Gerardus Mercator, a 16th-century Belgian cartographer, created one of the earliest solutions, the Mercator projection, which is still used today.
- Several other map projections have been developed, each with advantages and drawbacks.
- Three most useful map projection types, including Mercator’s, are commonly focused on.
POLAR PROJECTION
- The polar projection (Figure 1.10) centers on either the North or South Pole.
- Meridians appear as straight lines radiating from the pole.
- Parallels are concentric circles centered on the pole.
- Typically shows only one hemisphere, with the Equator as the map’s outer edge.
- Intersections of parallels and meridians form true right angles.
- Shows true shapes of small areas, maintaining correct shapes regardless of location on the map.
- Scale fraction increases outward, causing areas near the edge to appear larger than those near the center.
MERCATOR PROJECTION
- In the Mercator projection, meridians form a rectangular grid of straight vertical lines, and parallels form straight horizontal lines (Figure 1.11).
- Meridians are evenly spaced, but spacing between parallels increases with latitude, doubling at 60° compared to the Equator.
- Map must be cut off at an arbitrary parallel, such as 80° N, due to increased spacing near poles.
- Enlarges features near the poles.
- Designed for navigational purposes: a straight line on the map represents a line of constant compass direction.
- Navigators can draw a line between two points, measure the bearing relative to a meridian, and follow that compass direction.
- Does not show the shortest distance between two points accurately.
- Used to display straight-line features like flow lines of winds and ocean currents, directions of crustal features, and lines of equal values (e.g., temperature, pressure).
- Chosen for maps of temperatures, winds, and pressures due to its properties.
CONFORMAL AND EQUAL-AREA MAPS
- The shape and area of a small feature (e.g., island, peninsula) change when projected from globe to map.
- Some projections preserve shape but alter area; these are conformal projections.
- Mercator projection is an example of a conformal projection (Figure 1.11).
- In Mercator projection, shorelines and shapes of continents are shown accurately.
- However, Mercator projection distorts land areas, enlarging them at higher latitudes.
- Equal-area projections display areas correctly but distort shapes.
- No projection can be both conformal and equal-area; only a globe can preserve both properties.
WINKEL TRIPEL PROJECTION
- Winkel Tripel projection (Figure 1.12) invented by Oswald Winkel.
- “Tripel” refers to minimizing distortions to area, distance, and direction.
- Parallels are nearly straight, curving slightly toward edges.
- Meridians increasingly curved with distance from the central meridian.
- Neither perfectly conformal nor perfectly equal-area.
- Shapes of coastlines and continents distorted by shearing, increasing away from the central meridian and toward the poles.
- Areas of continents and countries more accurate than in Mercator projection.
- Polar regions near the edges have significant area distortion.
- Suitable for displaying global data with minimal distortion.
- Ideal for world maps showing climate, soils, and vegetation.
- Maps are efficient for compiling and storing spatial information.
- Geographic information systems (GISs) supplement maps for acquiring, storing, processing, analyzing, and outputting spatial data.
Global Time
- Time zones originated due to the Earth’s rotation and the need to standardize time across regions.
- Earth rotates 360 degrees in 24 hours, so each hour corresponds to 15 degrees of longitude.
- Solar noon, when the Sun reaches its highest point in the sky, occurs at different times for places with different longitudes.
- Time zones were established based on longitudinal divisions to synchronize clocks within a region.
- Each time zone typically spans 15 degrees of longitude, with adjustments for political boundaries and practical considerations.
- Differences in time between adjacent time zones are generally one hour apart, except in cases like Newfoundland (30 minutes offset from Atlantic Time).
- The International Date Line is located roughly along the 180 degrees longitude to avoid crossing time zones irregularly.
STANDARD TIME
- Standard time system divides the globe into time zones to simplify global timekeeping.
- Each time zone is typically aligned with a standard meridian, usually 15 degrees of longitude apart.
- Adjacent time zones differ by one hour in most cases; exceptions include Newfoundland, which is 30 minutes ahead of Atlantic Time.
- Figure 1.15 illustrates the time zones observed in northern North America, showing how regions are grouped into different time zones based on their longitudinal position.
- This system ensures that neighboring cities and countries can coordinate schedules and activities despite being in different longitudes.
WORLD TIME ZONES
- Russia spans 11 time zones from east to west but organizes them into 8 standard time zones.
- China covers 5 time zones but maintains a single national time based on the Beijing standard meridian.
- India and Iran use time offsets that depart from neighboring countries by 30 or 90 minutes, based on meridians positioned between standard meridians.
- Some regions, like Newfoundland in Canada and certain Australian states (South Australia, Northern Territory), use time zones based on 71⁄2° meridians.
- Time zones are often referenced by numbers indicating the hours ahead (+) or behind (-) Greenwich Mean Time (GMT). For instance, -7 indicates seven hours behind GMT, while +13 indicates thirteen hours ahead of GMT.
INTERNATIONAL DATE LINE
- The 180th meridian serves as the International Date Line (IDL).
- East of the IDL, it is one day earlier than west of it.
- It zigzags to avoid countries and territories, especially around island groups and New Zealand.
- Air travelers crossing the IDL may experience calendar shifts of a day forward (westward travel) or backward (eastward travel).
- The IDL is not a straight line and deviates for practical reasons, ensuring it avoids populated areas and aligns with political boundaries.
- It is crucial for global timekeeping and ensuring consistency in international travel scheduling.
DAYLIGHT SAVING TIME
- Daylight saving time (DST) involves setting clocks ahead by one hour (sometimes two) during part of the year.
- Originally aimed at saving energy and reducing accidents and crime, modern benefits are primarily economic, facilitating more retail shopping and recreational activities.
- In the United States, DST starts on the second Sunday in March and ends on the first Sunday in November.
- Several regions within the United States and territories, such as Arizona (except Navajo Nation), Hawaii, Puerto Rico, and others, do not observe DST.
- Internationally, many countries observe DST but start and end dates vary.
- In the European Union, DST is referred to as summer time, starting on the last Sunday in March and ending on the last Sunday in October.
PRECISE TIMEKEEPING
- Since the 1950s, atomic clocks have been the most accurate timekeeping devices, based on cesium atoms emitting microwave energy.
- Atomic clocks are incredibly precise, keeping time to better than one part in 1 trillion.
- Atomic time, independent of Earth’s rotation, serves as the universal standard.
- Coordinated Universal Time (UTC) is derived from atomic time and is used globally for civil timekeeping.
- UTC ensures a day remains 86,400 seconds (24 hours) to match Earth’s mean rotation rate relative to the Sun.
- UTC is managed by the Bureau International de l’Heure near Paris.
- Earth’s natural timekeeping varies slightly due to changes in its rotation speed and orbital variations, necessitating periodic adjustments to maintain synchronization with UTC.
The Earth’s Revolution Around the Sun
- Earth’s movement around the Sun is known as revolution, completing a full orbit in approximately 365.242 days.
- The calendar year of 365 days requires a leap year every four years to accommodate the extra time, adding a 29th day to February.
- Further adjustments, such as omitting leap days in century years not divisible by 400, help keep the calendar accurate.
- Earth’s orbit around the Sun is elliptical, with variations in distance; closest approach (perihelion) occurs around January 3, while farthest point (aphelion) is around July 4.
- Despite being elliptical, Earth’s orbit is nearly circular, with distance variation of about 3% over one revolution.
- From a vantage point above the North Pole, Earth revolves counterclockwise around the Sun, the same direction as its rotation on its axis.
MOTIONS OF THE MOON
- The Moon rotates on its axis and orbits the Earth in the same direction as Earth’s rotation around the Sun.
- Due to synchronous rotation, one side of the Moon (the near side) always faces Earth, while the far side remained unseen until 1959.
- The phases of the Moon depend on its position relative to the Earth and Sun, determining how much of the sunlit side is visible from Earth.
- It takes approximately 29.5 days for the Moon to complete its cycle from one full Moon to the next (lunar month).
- The appearance of the Moon in phases is due to varying angles of sunlight falling on its spherical surface, visible in Figure 1.19 as a half-full Moon with the Sun just below the horizon.
TILT OF THE EARTH’S AXIS
- Seasons occur because Earth’s axis is tilted relative to its orbit around the Sun, known as the plane of the ecliptic.
- The axis remains pointed towards Polaris (North Star) throughout Earth’s orbit, maintaining its orientation.
- As Earth orbits the Sun, different hemispheres receive varying amounts of direct sunlight due to the axial tilt.
- Summer occurs when a hemisphere tilts towards the Sun, leading to longer days and more direct sunlight.
- Winter happens when the hemisphere tilts away from the Sun, resulting in shorter days and less direct sunlight.
- Near the Equator, seasonal changes in daylight are minimal due to its proximity to the plane of the ecliptic, where sunlight distribution remains relatively consistent throughout the year.

THE FOUR SEASONS
- December 22 marks the December solstice (winter solstice in the northern hemisphere), when the North Pole tilts 23.5° away from the Sun, resulting in shorter days and winter.
- During the December solstice, the southern hemisphere experiences summer due to its tilt towards the Sun.
- Six months later, on June 21, the June solstice (summer solstice in the northern hemisphere) occurs when the North Pole tilts 23.5° towards the Sun, leading to longer days and summer in the northern hemisphere.
- At the June solstice, the southern hemisphere experiences winter as it tilts away from the Sun.
- Equinoxes, occurring around March 21 (vernal equinox in the northern hemisphere) and September 23 (autumnal equinox), see Earth’s axis not tilted towards or away from the Sun.
- Equinoxes result in equal lengths of day and night across the globe, marking the transitions between seasons.
- Dates of solstices and equinoxes can vary slightly each year due to Earth’s orbit not being exactly 365 days, requiring periodic adjustments in the calendar.
EQUINOX CONDITIONS
- Circle of illumination: Divides Earth into day and night hemispheres, always present due to Earth’s rotation.
- Subsolar point: Location on Earth’s surface where the Sun is directly overhead at a given moment.
- Equinox: Circle of illumination passes through North and South Poles, subsolar point is on the Equator.
- At equinox, poles receive minimal solar energy as Sun’s rays graze their surfaces.
- Noon angle: Angle at which Sun’s rays strike Earth’s surface at noon; equals 90° minus latitude.
- Example: At 40° N latitude during equinox, noon angle is 50°.
- Equal length of day and night (12 hours each) worldwide during equinox due to symmetrical illumination across the globe.
SOLSTICE CONDITIONS
- June solstice: At 40° N latitude, day is longer (about 15 hours) than night (about 9 hours).
- Equator during solstice: Equal day and night lengths (12 hours each) throughout the year.
- Arctic Circle (north of 66.5° N): Experiences continuous daylight (24 hours) during June solstice.
- Subsolar point during June solstice: Tropic of Cancer (23.5° N), where Sun is directly overhead.
- December solstice: At 40° N latitude, night is longer (about 15 hours) than day (about 9 hours).
- Antarctic Circle (south of 66.5° S): Experiences continuous daylight (24 hours) during December solstice.
- Subsolar point during December solstice: Tropic of Capricorn (23.5° S), where Sun is directly overhead.
- Twilight: Period when Sun is just below the horizon, providing illumination; longer at higher latitudes during polar night.
- Solstices and equinoxes are unique events marking changes in day length and Sun’s position relative to Earth’s axis.
- Subsolar point’s latitude changes throughout the year, oscillating between Tropic of Cancer and Tropic of Capricorn, known as Sun’s declination.