Nature of Geomorphology
Chapter – 1
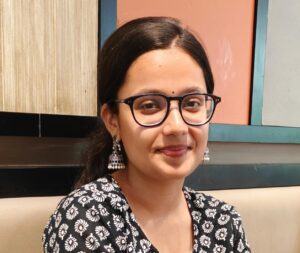
- Geomorphology has rapidly evolved in methodology and approaches since 1945.
- R.E. Horton introduced quantitative methods for analyzing morphometric characteristics of fluvially originated drainage basins in 1945.
- A clear division in the discipline emerged post-1950:
- Evolutionary approach: Focuses on progressive changes in landforms over long periods.
- Process-response approach: Focuses on the equilibrium model and steady state of landform development.
- There is a need to integrate different concepts in geomorphology:
- Cyclic concept: Involves long-term historical evolution of landforms.
- Non-cyclic concept: Involves dynamic equilibrium, functional, and process-response models.
- Micro-geomorphology: Focuses on smaller spatial and temporal scales.
- Mega-geomorphology: Focuses on larger spatial and longer temporal scales.
DEFINITION OF GEOMORPHOLOGY
- Geomorphology, oceanography, climatology, and biogeography are key fields in geography.
- The term “geomorphology” is derived from three Greek words: “ge” (earth), “morphe” (form), and “logos” (discourse).
- Geomorphology is the science of describing various forms of the earth’s surface, specifically topographic or geometric features (relief features).
- P.G. Worcester (1940) defined geomorphology as the interpretative description of the relief features of the earth’s surface.
- W.D. Thornbury (1954) included submarine forms in addition to surface reliefs within geomorphology.
- Geomorphology involves the scientific study of the earth’s surface features, their origin, development, and the processes that evolve these landforms.
- Easterbrook (1969) stated that all landforms are related to particular geologic processes, evolving over time through a sequence of forms depending on the duration of the processes.
- A.L. Bloom (1979) defined geomorphology as the systematic description and analysis of landscapes and the processes that change them.
SCOPE OF GEOMORPHOLOGY
- The subject matter of geomorphology can be organized based on:
- Dimension and scale of relief features (landforms).
- Processes that shape the landforms.
- Approaches to geomorphic studies.
- Geomorphology, as the study of landforms, has a well-defined framework.
- A systematic study of landforms requires fundamental knowledge of geology.
- The genesis and development of landforms primarily depend on the materials (geomaterials or structure) of the earth’s crust and partly on endogenetic forces.
- Geomorphology is sometimes equated with geology (W.D. Thornbury, 1954) and sometimes considered a branch of geology (A.K. Lobeck, 1939).
- Geomorphology originated from geology and is still often housed in geology departments in many American universities.
- Some aspects of geology, such as structural and dynamic geology, are included in the description and analysis of landforms.
- Theoretical geology helps in understanding the nature of landforms and the origin of different types of reliefs like mountains, plateaus, continents, and ocean basins.
- Endogenetic forces, particularly diastrophic and sudden forces (vulcanicity and seismic events), introduce irregularities on the earth’s surface, generating variety in landforms.
- Based on dimension and scale, the relief features of the earth’s surface, the core subject matter of geomorphic study, can be grouped into three broad categories of descending order.
1)RELIEF FEATURES OF THE FIRST ORDER
- “On the smallest scale and covering the largest area is world geomorphology” (C.A.M. King, 1966).
- World geomorphology includes the consideration of continents and ocean basins.
- The description and analysis of worldwide erosion surfaces require the consideration of the characteristics and evolution of continents and ocean basins.
- Continents and ocean basins are considered the relief features of the first order.
- The analysis of continental drift, caused by forces from within the earth (thermal convective currents) or outer sources (tidal forces, gravitational forces, etc.), is essential for understanding major morphological features of the earth’s surface.
- Plate tectonics are crucial for understanding the origin of continents and ocean basins.
2)RELIEF FEATURES OF THE SECOND ORDER
- The structural forms developed over a continent or part thereof (e.g., mountains, plateaus, lakes, faults, rift valleys) constitute the category of relief features of the second order.
- These forms owe their genesis mainly to endogenetic forces, particularly diastrophic forces.
- The nature, mode, and rate of operation of these endogenetic forces must be studied properly.
- Proper study ensures that the general characteristics, nature, and mode of origin of the second order relief features are well understood.
- Second order relief features serve as the foundation upon which third order reliefs are produced.
- These second order features are referred to as constructional landforms
3)RELIEF FEATURES OF THIRD ORDER
- Micro-level landforms developed on second order relief features by exogenetic denudational processes originating from the atmosphere are included in the third order relief features.
- These landforms can be:
- Erosional: e.g., glacial valleys, river valleys, karst valleys, cirques, canyons, gorges, terraces, yardangs, sea cliffs.
- Depositional: e.g., drumlins, eskers, flood plains, natural levees, deltas, sea beaches, sand dunes, stalactites, stalagmites.
- Residual: e.g., monadnocks, inselbergs or bornhardts.
- Minor tectonic features caused by endogenetic forces.
- Third order relief features are given more importance in geomorphic studies as they constitute the core subject matter of geomorphology.
- The nature, mode, and rate of operation of denudational processes, which produce third order relief features, are studied at varying spatial and temporal scales.
- Besides natural geomorphological processes, anthropogenic processes are also important in geomorphic investigations.
- Human activities, through economic and technological means, have significantly increased the rate of natural processes.
- The subject matter of geomorphology can also be organized based on geomorphic processes (both endogenous and exogenous) that shape the landforms and approaches to the study of landforms.
- The Davisian dictum states that “landscape is a function of structure, process, and time.”
- K.J. Gregory’s geomorphic equation (F=f (PM)dt, where F = landforms, f = function of, P = processes, M = geomaterials, dt = change over time) highlights the need for careful investigation of geomorphological processes, geomaterials, and the time factor.
- The dynamic equilibrium theory advocates for the exclusion of the time factor, arguing that landforms are time-independent.
- Geomorphic studies incorporate two major approaches:
- Historical studies: involve the historical evolution of landforms.
- Functional studies: involve time-independent series of landform evolution reflecting the association between landform characteristics and existing environmental conditions.
- Both approaches are relevant in geomorphological investigations.
EVOLUTION OF GEOMORPHOLOGICAL
THOUGHTS
- The present status of geomorphology is the result of gradual but successive development of geomorphic thoughts postulated over different periods by numerous philosophers, experts, and geoscientists.
- The developmental phases of geomorphology indicate its dynamic nature.
- Geomorphology originated from the philosophical ideas of the ancient Romans and Greeks.
- The subject developed through the methodological contributions of the 18th and 19th centuries.
- Geomorphology reached its golden status in the 1st and 2nd decades of the 20th century with the postulation and wider acceptance of the cyclic concept of landscape development and denudation chronology.
- After 1950, major changes occurred in the methodological aspects of geomorphology:
- Rejection of the Davisian model of cyclic development of landforms.
- Introduction of quantitative methods in geomorphological studies.
- Postulation of the dynamic equilibrium theory of landscape development, based on the concept of time-independent series of landform evolution.
- Increased emphasis on process geomorphology (process-response model).
- Emergence of environmental geomorphology.
- Shift from mega-geomorphology to micro-geomorphology.
- Shift from longer temporal scales to shorter temporal scales.
- Increased focus on the applied aspects of the subject.
1) ANCIENT PERIOD
- Geomorphology has developed from the work of late eighteenth and nineteenth century geologists and hydrologists (C.A.M. King, 1966).
- Some ideas regarding landforms were indirectly postulated even in ancient times by philosophers and historians of Greece, Rome, Egypt, etc.
- Herodotus (485 B.C.—425 B.C.), a noted Greek historian, made significant contributions to understanding rivers’ alluvial behavior during his extensive travels in Egypt.
- Observed the depositional work of the Nile and postulated that “Egypt was the gift of the Nile.”
- Related the shape of the depositional feature at the mouth of the river to the Greek letter Δ (Delta) and named it delta.
- Postulated the concept of the gradual growth of the delta towards the sea.
- Inferred from marine fossils in the Nile’s alluvium far inland that the sea level is not permanent and undergoes transgressional (advance) and regressional (retreat) phases.
- Aristotle (384 B.C.—322 B.C.), a reputed Greek philosopher, presented interesting ideas regarding water springs, origin of streams, and the behavior of seas and oceans.
- Suggested that spring-fed streams are seasonal and ephemeral (non-permanent).
- Stated that limestones cannot maintain permanent surface drainage as most streams disappear to form subterranean drainage.
- Proposed that water springs are supplied by rainwater (via percolation and seepage), condensation of underground saturated air, and water vapor.
- Was aware of the changing nature of sea levels and the deposition of eroded materials by rivers as alluvium.
- Strabo (54 B.C.—25 A.D.), a noted historian, made significant contributions regarding the depositional work of rivers.
- Suggested that the size and shape of deltas depend on the nature of the terrain through which a river flows.
- Noted that extensive regions with weaker rocks produce larger deltas due to higher sediment yield, while regions with resistant rocks maintain smaller deltas due to less erosion and sediment production.
- Inferred the concept of differential erosion from his statements.
- Seneca maintained that “rivers deepen their valleys through abrasion.”
- Ancient philosophers and historians forwarded some incoherent ideas, but they could not collectively reach any definite conclusion.
2) DARK AGE
- With the fall of the Roman Empire, there was a lull in the development of geographical and geomorphological thoughts for a long period of 1400 years (from the 1st century A.D. to the 14th century A.D.).
- During this period, a few glimpses of geomorphological ideas were put forth by thinkers such as Avicenna (980—1037 A.D.), an Arabian thinker.
- Avicenna proposed that mountains should be divided into two categories:
- Mountains originated due to upliftment.
- Mountains originated due to erosion by running water.
3) AGE OF CATASTROPHISM
- The long academic silence of 1400 years was suddenly broken by the emergence of catastrophists.
- Catastrophists believed in the quick and sudden origin and evolution of all animate and inanimate objects in a very short period of time.
- This belief added new and peculiar concepts to geomorphological and geographical literature.
- The age of the earth was calculated to be a few thousand years by the catastrophists.
- Only events that occurred within the lifetime of people were given cognizance.
- Sudden endogenetic forces like volcanic eruptions and earthquakes likely influenced these thinkers to postulate such fantastic and unrealistic ideas.
- These ideas were not only related to landforms but to all animate and inanimate objects.
- The concept of sudden change and evolution also influenced biologists, who believed in the sudden evolution and destruction of all living organisms.
4) AGE OF UNIFORMITARIANISM
- The concept of catastrophism was finally rejected by James Hutton (1726—1797 A.D.) in the 18th century A.D., who postulated the gradual cyclic nature of Earth’s history through his concept of uniformitarianism.
- Geomorphic and geological thoughts began to gain coherence in the 15th, 16th, and 17th centuries.
- Leonardo da Vinci (1452—1519 A.D.) proposed that rivers formed their valleys through vertical erosion and deposited eroded materials elsewhere.
- Buffon (1707—1788 A.D.) rejected catastrophist ideas about the earth’s age being only thousands of years old. He emphasized rivers as powerful agents of erosion capable of reducing uplifted land to sea level.
- Targioni Tozzetti (1712—1784 A.D.), an Italian thinker, postulated that the irregular courses of rivers (symmetry and asymmetry of valleys) depended on the nature of rocks they flowed through. This concept hinted at differential erosion.
- Guettard (1715—1786 A.D.) noted that not all eroded sediments from rivers are deposited in seas; some are deposited as flood plains along river courses. He also recognized the erosive power of marine processes.
- Dimarest (1725—1815 A.D.) proposed that rivers themselves formed the valleys they flow through, deepening them over time. He introduced the concept of landform development through successive stages.
- James Hutton’s concept of uniformitarianism in the 18th century emphasized that the same geological processes observed today operated in the past, leading to cyclic patterns in Earth’s history.
- Hutton’s work was first published as a research paper titled “Theory of the Earth” in 1788 and later expanded into a book in 1795.
- John Playfair (1748—1819 A.D.), a close friend of Hutton, elaborated on uniformitarianism in his book “Illustrations of the Huttonian Theory of the Earth” (1802), emphasizing fluvial and glacial processes.
- Charles Lyell (1797—1873 A.D.), influenced by Hutton, laid the foundation of modern historical geology. He defined geology as the science investigating successive changes in both organic and inorganic kingdoms.
- Lyell’s major works include “Principles of Geology” (in two volumes) and “The Geological Evidences of the Antiquity of Man” (1863).
- C.G. Greenwood (no dates provided) contributed significantly with his paper “Rain and Rivers: or Hutton and Playfair against Lyell and all comers” (1857), promoting the concept of base-level of erosion before Powell in America
5) MODERN AGE (NINETEENTH CENTURY)
- Geomorphology emerged as an independent discipline and major branch of geology in the early 19th century, leading to the development of two distinct schools of thought: the European School and the American School.
- European School:
- Emphasized the recognition and identification of Pleistocene Ice Age and glaciation, marine erosion, fluvial processes, arid and karst landscapes.
- Sir Charles Lyell (1797—1873 A.D.) endorsed and popularized James Hutton’s concept of uniformitarianism through his influential books “Principles of Geology” (two volumes).
- Lyell’s contributions laid the groundwork for Charles Darwin’s “Origin of Species”. His book “The Geological Evidences of the Antiquity of Man” (1863) incorporated many of Hutton’s concepts.
- Louis Agassiz (1807—1873 A.D.) is credited with early recognition of the Pleistocene Ice Age, presenting his ideas in 1840. He identified thick continental glaciers covering northern Europe during this period.
- James Geikie, a Scottish geologist, published “The Great Ice Age” in 1894, elaborating on multiple glacial and interglacial periods within the Ice Age.
- A. Penck and E. Brückner identified four glacial periods (Gunz, Mindel, Riss, Wurm) during the Pleistocene Ice Age based on observations over the Alps.
- In marine erosion, attention was focused on corrasion by sea waves. Sir Andrew Ramsay (1814—1891) described marine platforms formed by marine erosion in Wales and S.W. England.
- Baron Ferdinand von Richthofen (1833—1905) contributed significantly to understanding marine erosion during his studies in China, supporting a marine origin for plains formed beneath marine transgressions.
- C.G. Greenwood, a British geologist, made notable contributions in subaerial erosion and was among the first to propose the concept of base level of erosion, preceding Major Powell in the U.S.A.
- Jukes (1862) categorized rivers into transverse streams (flowing across geological structures) and longitudinal streams (following rock bed directions), proposing that longitudinal streams are subsequent to transverse streams.
American School:
- Considered the pinnacle of geomorphological contributions, particularly during the late 19th and early 20th centuries (1875-1920), known as the “golden age” of American geomorphology and worldwide.
- William Morris Davis (1850-1934) introduced the concept of landscape development theory, which became fundamental in geomorphology. His ideas centered around the geographical cycle of erosion, emphasizing sequential changes in landforms over time.
- Major J.W. Powell (1834-1902) conducted extensive studies of the Colorado Plateau and Uinta Mountains. He proposed the geological structure as the basis for classifying landforms and introduced the concept of base level, defining it as the limit of maximum vertical erosion by streams determined by sea-level.
- C.A. Malott (1928) further classified base levels into ultimate, local, and temporary, and inferred the concept of peneplain, an erosional level plain slightly above sea level resulting from prolonged fluvial erosion.
- Powell also categorized river valleys into antecedent, superimposed, and consequent valleys based on their evolutionary stages.
- G.K. Gilbert (1843-1918) is regarded as the first true geomorphologist in America. He contributed significantly to systematic and quantitative geomorphology, stressing the importance of creative imagination and testing hypotheses.
- Gilbert formulated several laws including the law of uniform slope, law of structure, and law of divides. He introduced the concept of graded profiles of rivers and established relationships among load, volume, velocity, and channel gradient through quantitative analyses.
- C.F. Dutton (1843-1912) introduced the term isostasy to describe the equilibrium condition of the Earth’s upstanding and downstanding landmasses. His studies of the Colorado Plateau and Grand Canyon provided evidence supporting Powell’s base level concept and emphasized the role of prolonged fluvial erosion in canyon formation.
- W.M. Davis (1850-1934), Professor of physical geography at Harvard University, considered the patron of geomorphology due to his extensive contributions and direction to the study of landforms.
- Davis systematically integrated scattered ideas of American geomorphologists into a coherent framework, leading to the recognition of the American school of geomorphology as the Davisian school.
- Credited with formulating the first general theory of landscape development, which synthesized his major concepts: complete cycle of river life (1889), geographical cycle (1899), and slope evolution.
- The concept of the complete cycle of river life emphasized the progressive development of erosional stream valleys.
- Introduced the geographical cycle to highlight sequential changes in landscapes over time, proposing that landforms undergo cyclic development under uniform external environmental conditions.
- According to Robert C. Palmquist, Davis’s theory posits that landforms change in an orderly sequence as processes operate through time.
- Further details on Davis’s contributions to geomorphological thought will be elaborated in the subsequent sections, particularly in Chapter 3 of this book.
6) MODERN AGE (20TH CENTURY : FIRST HALF)
- Initiated a methodological revolution in geomorphological studies at the beginning of the 20th century, alongside his followers in the USA and abroad, including W. Penck in Germany.
- Davis’s classical model of the geographical cycle, introduced in 1899, described as a period during which an uplifted landmass transforms through land sculpturing processes into a low, featureless plain known as a peneplain.
- Despite opposition, Davis’s geographical cycle dominated geomorphological investigations worldwide in the first half of the 20th century, under various names such as normal cycle, erosion cycle, geomorphic cycle, and humid cycle.
- His general theory of landscape development posited sequential changes in landforms through successive stages, aiming towards the attainment of a peneplain.
- Identified three fundamental factors controlling landform evolution: structure, process, and time, collectively referred to as the ‘trio of Davis’.
- Extended his geographical cycle concept beyond fluvial processes to include other erosional processes: arid cycle of erosion (1903, 1905, 1930), glacial cycle of erosion (1900, 1906), marine cycle of erosion (1912 by Davis, 1919 by D.W. Johnson), karst cycle of erosion (Beede 1911, Cvijic 1918), periglacial cycle of erosion (L.C. Peltier 1950).
- Davis’s concept of historical evolution of landscapes formed the foundation for classical denudation chronology and erosion (planation) surfaces in the UK.
- Published his major contributions in a book titled ‘Geographical Essays’ in 1909, showcasing his skills as a definer, analyzer, interpreter, systematizer, and synthesizer of geomorphological knowledge.
- Revered by peers and successors alike for his academic prowess and rhetorical style, Davis remains a towering figure in the advancement of geomorphological understanding.
D.W. Johnson contributed significantly to marine processes and coastal geomorphology within the American school of geomorphology.
C.A. Malott made notable contributions to the study of fluvial processes and erosion, further enriching the American school’s understanding.
H.A. Mayerhoff and E.W. Olmsted studied the evolution of Appalachian drainage systems, adding to the knowledge base of American geomorphology.
R.P. Sharp, C.P.S. Sharp, A.K. Lobeck, W.D. Thornbury, etc., each contributed distinctively to various branches of geomorphology, enhancing the American school’s breadth of expertise.
The European school of geomorphology, particularly in the first half of the 20th century, developed an independent identity focused on chronological studies of landscape development, known as denudation chronology.
S.W. Wooldridge and J.A. Steers were notable British geomorphologists during this period, contributing influential works that shaped understanding in their respective fields.
German geoscientists critiqued Davis’s cyclic concept of landform evolution, with some advocating for outright rejection and others proposing modifications or new models. W. Penck argued for a time-independent approach through morphological analysis.
Climatic geomorphology emerged in France and Germany, positing that different climatic types produce distinct landform assemblages. Key figures like Sauer, Wentworth, and Saper laid the groundwork, with Budel and Peltier advancing morphoclimatic regions.
The quantitative revolution in geomorphology began with statistical techniques introduced by Krumbein and was further propelled by R.E. Horton’s morphometric analyses of fluvial drainage basins.
Post-1940s, there was increasing criticism of Davisian evolutionary models and descriptive geomorphology, leading to calls for alternative theories emphasizing time-independent series of landforms.
L.C. Peltier’s introduction of the periglacial cycle of erosion in 1950 provided new support for Davisian cycle models amid evolving geomorphological theories.
7) RECENT TRENDS (SECOND HALF OF 20TH CEN
TURY)
Post-1950, geomorphology witnessed increasing criticism of Davisian cyclic models and a shift towards dynamic equilibrium theories emphasizing time-independent landscape evolution.
J.T. Hack and R.J. Chorley pioneered the dynamic equilibrium theory, viewing landscapes as open systems in steady-state balance due to continuous energy and material inputs and outputs.
Hack’s model highlighted geological controls on landform evolution, balancing geomaterial resistance with erosive processes, albeit acknowledging evolutionary possibilities.
Despite the rejection of cyclic models, new theories like geomorphic threshold, tectonic-geomorphic, and episodic erosion models emerged to address conceptual gaps.
Quantitative geomorphology became dominant, employing deductive methods and field instrumentation to study landforms and processes at micro-spatial and temporal scales.
Process geomorphology replaced descriptive approaches with a focus on understanding form-process relationships through quantitative data and field measurements.
System theory was introduced to explain landforms and processes, with models like natural analogue, physical systems, and general systems aiding in geomorphic analyses.
Environmental geomorphology emerged as a significant branch of applied geomorphology, addressing environmental issues caused by human interactions with geomorphological processes.
Monitoring and study within environmental geomorphology help in assessing and mitigating impacts such as erosion, sedimentation, and soil loss in man-impacted areas.
INDIAN CONTRIBUTIONS TO GEOMORPHOLOGY
Geomorphological research in India commenced relatively late due to delayed establishment of postgraduate geography programs, with significant milestones including Aligarh Muslim University in 1931, Calcutta University in 1941, and others in subsequent years.
Early contributions in Indian geomorphology were sporadic and derived from reports, articles, and essays by administrators, travelers like Swami Pranawanand, and geologists such as Heren, Wadia, Dunn, and others.
The Davisian model of the cycle of erosion and denudation chronology formed the basis of early Indian geomorphological studies, utilizing data primarily from topographical maps and gazetteers.
The 1950s and 1960s marked a significant period of growth in Indian geomorphological research, focusing on various physiographic regions of peninsular India with notable contributions from researchers like R.P. Singh, A.K. Sen Gupta, E. Ahmad, and others.
The 21st International Geographical Congress held in New Delhi in 1968 spurred increased interest and systematic studies in Indian geomorphology across different regions of the country.
Systematic studies included research on floods in the Mahanadi River by B.C. Acharya, landform evolution in the Lower Son Valley by G.K. Datta, and glacial landforms by M.K. Bandopadhyay, among others.
Morphometric techniques using topographical maps were pioneered by R.L. Singh in 1967, laying the foundation for detailed terrain analysis and subsequent doctoral dissertations at institutions like Banaras Hindu University.
Post-1968, Indian geomorphological research expanded significantly, encompassing fluvial, arid, glacial, coastal, structural, and quantitative geomorphology, employing advanced morphometric methods.
Noteworthy contributions included studies on the evolution of drainage patterns in Goa, erosion surfaces on the Rewa Plateau, and the classification of terrain characteristics across various regions of India.
The systematic recognition of drainage basins as ideal geomorphic units led to the initiation of systematic morphometric analysis, notably starting with Renu Srivastava’s doctoral thesis on the Belan River at Allahabad University in 1976.
Subsequent to Srivastava’s work, several doctoral theses were presented at Allahabad University, focusing on small drainage basins of various regions such as the Ranchi Plateau, Palamau Upland, and S.E. Chotanagpur.
The 1980s and 1990s marked a period characterized by a deeper exploration into the causal relationships between landforms and processes, leading to the formulation of models and techniques in Indian geomorphology.
Significant theoretical contributions included Savindra Singh’s estimation of drainage density based on drainage texture and Anil Kumar’s field-based study on residual hills in the Jamalpur-Kiul Hills, published in Zeitschrift fur Geomorphologie.
International publications during this period included works by H.S. Sharma, R.K. Rai, and Savindra Singh and R.S. Pandey, focusing on topics such as climate and drainage morphometric properties and morphological analysis over Bhander Scarps.
V.S. Kale’s publication on the Western Ghats and Savindra Singh’s research on rill and gully erosion in the subhumid tropical riverine environment of Teothar Tahsil, M.P., contributed significantly to field and laboratory-based geomorphological studies.
The establishment of the Indian Institute of Geomorphologists at Allahabad University following the International Conference on Geomorphology and Environment in 1987 boosted geomorphological research in India, particularly in river-bed morphology, alluvial morphology, and coastal geomorphology.
The Allahabad Centre of Geomorphology, established in 1971, intensified its research efforts in the 1980s and 1990s, focusing on environmental geomorphology, fluvial geomorphology, and urban geomorphology, with notable contributions from researchers like Alok Dubey and Savindra Singh.
Significant doctoral theses during this period included studies on solution topography of Rohtas Plateau and applied geomorphology of the Belan-Son interstream area, demonstrating diverse applications of geomorphological knowledge.
Research projects funded by DST, New Delhi, such as the study on ‘gully erosion and management’ in a micro-man-impacted gully basin, further advanced the understanding of meteorological, hydrological, and geomorphological variables in erosion processes.
The Poona Centre of Geomorphology is renowned for its extensive research in various fields including fluvial geomorphology, structural geomorphology, river bed morphology, alluvial geomorphology, and coastal geomorphology.
Initiated by K.R. Dikshit, the centre encouraged young geomorphologists to conduct field instrumentation of processes, leading to significant contributions from researchers like V.S. Kale and S.R. Jog in fluvial geomorphology.
Numerous research projects funded by organizations such as U.G.C., D.S.T., and others have been undertaken by K.R. Dikshit, V.S. Kale, and S.R. Jog, further enriching the field of geomorphology.
S.N. Karlekar and associates have focused extensively on the western coasts of Maharashtra, making notable contributions to coastal geomorphology, while S.R. Basu has monitored landslides in the eastern Himalaya region.
The Calcutta Centre of Geomorphology, credited with an early start in geomorphological research, owes its development to pioneers like S.P. Chatterjee and K. Bagchi. Presently, the Department of Geography at Calcutta University is well-known for research across various branches of geomorphology.
M.K. Bandopadhyay’s active engagement in studying glacial geomorphology in the Himalayas, including regular monitoring of glacier recession through field studies, highlights significant contributions from this centre.
S.C. Mukhopadhyay has made substantial contributions in fluvial geomorphology, while landslides in the eastern Himalayas have been systematically monitored under his supervision.
The geomorphologists at the Central Arid Zone Research Institute (CAZRI), Jodhpur, including Bimal Ghose, Surendra Singh, P.C. Vats, and Amalkar, have conducted outstanding research in arid geomorphology and applied geomorphology based on intensive field surveys and remotely sensed data.
R.K. Rai and colleagues are actively engaged in various facets of geomorphology at Shillong, focusing on fluvial geomorphology, structural geomorphology, and karst geomorphology.
Geomorphological research is also vibrant at locations such as Bhagalpur (led by Anil Kumar), Jaipur (H.S. Sharma), Delhi (S.K. Pal), Jammu (M.N. Kaul), Thanjavur (Victor Raja Manickam), Almora (J.S. Rawat and R.K. Pandey), Varanasi (K. Prudhvi Raju), and Srinagar-Garhwal (Devidatt).
An outstanding contribution to the field includes the development of computer software for interpreting satellite imagery for geomorphological purposes, spearheaded by S.R. Jog from Pune.
SYSTEM CONCEPT
- Geomorphic systems integrate various landforms and processes under specific conditions of energy input (e.g., precipitation, insolation) and output of matter.
- The balance between energy input and matter output is crucial for the effective functioning of geomorphic systems.
- According to Chorley, Schumm, and Sudden (1985), geomorphic systems consist of interacting processes and landforms that collectively shape landscapes.
- These systems can exist in steady states, dynamic equilibrium, or undergo changing states over time.
- Super geomorphic systems comprise interconnected subsystems with diverse landform suites, linked by input-output interactions.
- Geomorphic systems are classified into closed and open systems based on their boundary characteristics.
- Closed systems like the Davisian geographical cycle have fixed boundaries where neither energy nor matter crosses out, evolving through positive feedback mechanisms.
- Open systems, exemplified by drainage basins, continuously renew energy and expel matter, maintaining equilibrium via negative feedback.
- Feedback mechanisms (positive and negative) control the internal structure of geomorphic systems.
- Positive feedback amplifies changes caused by external inputs, leading to progressive changes in landforms over time.
- Negative feedback, or homeostasis, stabilizes geomorphic systems against external disturbances, maintaining equilibrium.
- An example of positive feedback: increased rainfall accelerates surface runoff, leading to soil erosion and eventually lowering relief.
- Negative feedback ensures equilibrium in streams: if sediment load increases due to erosion, the stream adjusts by depositing sediment until equilibrium is restored.
GEOMORPHIC MODELS
- Models are simplified approximations of the external real world, encompassing structured ideas, hypotheses, theories, or laws.
- They can manifest as roles, relations, equations, or synthesis of data, facilitating reasoning about the real world through spatial or historical translations.
- Characteristics of models include selective approximation of relevant aspects while disregarding detailed elements of the real world.
- Models are structured ideas that interconnect relevant aspects to project the real world in a simplified and generalized form.
- They are suggestive, allowing for future extension and generalization based on evolving knowledge and understanding.
- Models function as analogies, providing a framework for understanding complex phenomena in simpler terms.
- They possess reapplicability, enabling their use to analyze and predict real-world phenomena across various contexts.
- The functional roles of models encompass psychological aspects, aiding in visualizing and comprehending complex phenomena.
- They serve an acquisitive role by facilitating the acquisition of data, information, and ideas essential for model formulation.
- Models have a logical aspect, enabling geographers to explain data details and information coherently.
- Normatively, models allow for comparing selected phenomena with previously known situations, enhancing understanding.
- They serve a constructional role by providing the basis for formulating theories and laws to explain natural processes and patterns.
- Models are classified based on the familiarity of the situation and existing reality into:
- Descriptive models: Describe real situations with empirical information.
- Normative models: Describe less familiar situations based on more familiar ones.
- Models can be classified based on their form into:
- Hardware models, physical models, and experimental models.
- Theoretical models, symbolic models, and conceptual models.
- Models are also classified based on the system concept into:
- Synthetic system models.
- Partial system models.
- Black box models.
- According to R.J. Chorley (1967), conceptual geomorphic models can be approached in three ways:
- In terms of time and space.
- In terms of physical system.
- In terms of general system.
- Natural Analogue System:
- Describes geomorphological phenomena based on analogous natural systems that are simpler and better understood.
- Divided into historical natural analogue systems and spatial natural analogue systems.
- Historical natural analogue system:
- Considers time-controlled sequences where past geomorphic activities are repeated over time.
- Allows reconstruction of past geomorphic history based on present processes and resultant features.
- Spatial natural analogue system:
- Describes geomorphic features based on comparisons with identical and contiguous regions that are better understood.
- Involves describing one area based on another similar area known more comprehensively.
- Examples include Fenneman’s physiographic regions (1914), morphotectonic-based tectonic provinces, and climatic type-based morphogenetic regions.
- Physical System approach dissects geomorphic problems into component parts and studies their operations and interconnections.
- It presents a comprehensive synthesis of the entire physical system, integrating all component parts.
- Geomorphological investigations using the Physical System approach are based on quantitative methods.
- The Physical System includes three interconnected models:
- Hardware model: Simulates natural geomorphic complexes in the laboratory under controlled conditions, albeit on smaller spatial and temporal scales.
- Examples include developing river meanders or rills and gullies in laboratory settings.
- Challenges include the inherent complexity of natural geomorphic systems and issues related to spatial and temporal scales.
- Mathematical model: Abstract forms of equations where natural geomorphic phenomena, forces, processes, events, etc., are represented by mathematical variables, parameters, symbols, letters, and constants.
- For instance, K.J. Gregory’s mathematical formulation of Davisian landscape theory as the equation F = f(MP) t.
- Experimental design model: Focuses on designing experiments to understand specific geomorphic processes under controlled conditions.
- Hardware model: Simulates natural geomorphic complexes in the laboratory under controlled conditions, albeit on smaller spatial and temporal scales.
Mathematical models are classified into deterministic and stochastic types:
- Deterministic models establish predictable relationships between independent and dependent geomorphic variables. Examples include Horton’s laws of stream numbers and stream orders, which follow exponential function models.
- Stochastic models incorporate statistical aspects to account for random effects in natural processes, revealing simpler cause-and-effect relationships.
Experimental design models:
- These models identify meaningful components within observational data using structured experimental designs.
- They employ tools like regression analysis and harmonic analysis to analyze data and derive generalizations.
- Examples include A.N. Strahler’s models showing linear relationships between channel slope, discharge, and stream dimensions.
General system approach:
- Focuses on organized groups of geomorphic phenomena as a broad system.
- Emphasizes understanding the system’s overall operation rather than detailed study of individual components.
- Geomorphic systems like drainage basins exemplify this approach, functioning through inputs (solar energy, precipitation) and outputs (energy and matter).
- General system classified into synthetic system, partial system, and black boxes.
- Process-response model is culmination of synthetic system, built on structure and analysis of geomorphic events.
- Partial system aims to establish functional relationships between subsystems or factors in a geomorphic system.
- Detailed understanding of subsystems not essential; focus on interrelationships to predict system behavior.
- Black boxes require no detailed knowledge of internal structure of geomorphic components.
- Constructed based on assumptions rather than detailed process knowledge.
- Examples include G.K. Gilbert’s dynamic equilibrium model, climatic geomorphology by German and French geomorphologists, W.M. Davis’s geographical cycle, W. Penck’s morphological system.
- Geomorphic models must be practical for application purposes.
- Natural physical systems exhibit homeostatic mechanisms with negative feedback.
- Human interventions sometimes exceed system resilience, disrupting equilibrium.
- Geomorphologists should regulate interventions in landform systems using proven models to predict impacts accurately.
METHODS AND APPROACHES TO THE
STUDY OF LANDFORMS
- A geomorphologist’s main tasks include describing, classifying, and explaining erosional and depositional landforms.
- Description and explanation of landforms can be approached qualitatively or quantitatively, empirically or systematically, and regionally.
- Methods for analyzing landforms encompass inductive reasoning, deductive reasoning, and various analytical approaches.
- A system approach involves studying landforms as integrated systems, focusing on interactions and feedback mechanisms within these systems.
A) MAIN TASKS
(I) DESCRIPTION OF LANDFORMS
- ubjective description involves generalized and literary presentation of physical landscapes by non-specialists, lacking scientific rigor and objectivity.
- Genetic description goes beyond general information to explain the causes and processes behind landform development, such as erosion patterns or meander formation, often criticized for its lack of precision and explanatory depth.
- Objective description, also known as quantitative or scientific description, employs mathematical and statistical techniques. It involves precise measurements of landforms using fieldwork, topographical maps, air photographs, or satellite imagery. This approach is essential for morphometric analysis of landscapes and quantifying processes like river flow, sediment movement, weathering rates, and soil processes.
(II) CLASSIFICATION OF LANDFORMS
- Quantitative (non-genetic) classification relies on numerical data obtained through morphological mapping, field instrumentation, and interpretation of aerial imagery. It describes landforms based on observable features such as slope angles and plan forms without considering their origin or development processes.
- For example, hillslope profiles can be classified based on slope angles ranging from level slopes (0°—0.5°) to precipitous or vertical slopes (45°—90°). Drainage basins may be categorized by stream order (1st, 2nd, 3rd, etc.) and water flow periodicity (ephemeral, seasonal, perennial).
- Genetic classification categorizes landforms based on their mode of origin and development processes. It divides landform assemblages into categories such as tectonic slopes (due to faulting, folding), erosional slopes, and depositional slopes.
- Streams under genetic classification are categorized as sequent (following regional slope) or insequent (flowing across geological structures). Sequent streams further classify into consequent, subsequent, obsequent, and resequent streams, while insequent streams include antecedent and superimposed streams.
- Landform assemblages are also classified based on developmental stages like youthful, mature, and old landscapes as proposed by W.M. Davis, or cyclic origins such as mono-cyclic, poly-cyclic, rejuvenated, and exhumed landforms.
- Morphogenetic classification according to climatic types categorizes landscapes into humid, sub-humid, arid, semi-arid, and glacial types (W. Penck) or more detailed categories like glacial, periglacial, boreal, maritime, selva, moderate, savanna, semi-arid, and arid landscapes (L.C. Peltier).
(Ill) EXPLANATION OF LANDFORMS
The structure-form approach focuses on geological structure and lithological characteristics as dominant factors in shaping landforms like hillslopes, scarps, and karst topography.
Climatic geomorphology attempts to correlate specific landform types with different climate conditions, but struggles with identifying consistent diagnostic landforms for particular climates due to their occurrence in multiple climatic regions.
The historical or chronological approach employs uniformitarianism to describe the sequential changes in landforms over time, using concepts like Davisian cycles (youth, mature, old) to reconstruct denudation chronologies and interpret ancient erosion surfaces.
The process-form approach establishes relationships between geomorphic processes (weathering, erosion, deposition) and resultant landforms, employing scientific methods such as mathematical modeling and statistical analysis to study process rates and modes.
Critics of the process-form approach argue that geomorphology should focus on interpreting forms rather than studying processes, highlighting challenges such as the variable rates of geomorphological processes and the difficulty in isolating causal relationships between processes and landform features.
B) APPROACHES TO GEOMORPHOLOGICAL
ANALYSIS
- Geomorphic studies can be approached through a historical approach, focusing on the long-term evolution of large geographical areas over geological time scales.
- Alternatively, a functional approach examines landform characteristics in smaller areas over shorter periods, emphasizing the relationship with present-day processes.
- Descriptions of morphological characteristics on a larger scale can utilize a historical or chronological approach, tracing the sequential changes in landforms over time.
- An empirical approach provides a practical method to describe landform characteristics based on observed data and measurements without necessarily focusing on historical context.
- Landform characteristics can also be explained regionally, focusing on specific geographic regions and their unique morphological features.
- Conversely, a systematic approach generalizes landform characteristics across different regions, aiming to establish common principles or classifications applicable to various landscapes.
(I) HISTORICAL APPROACH
- The historical approach in landform studies focuses on describing the evolution of landforms over successive geological stages or cycles, spanning large spatial and temporal scales.
- It employs the concept of “palimpsest topography,” where the landscape bears imprints of past geomorphological processes overwritten by subsequent processes over time.
- This method reconstructs the past geomorphic history of a region using present and remnant landforms, following the principle of “present is key to the past.”
- Known as denudation chronology, its objective is to identify, date, and interpret ancient erosion surfaces and relic features based on drainage development and past tectonic events.
- Critics argue that this approach is highly deductive, relying on limited known information to describe unknown past events and responses.
- One major criticism is its limited explanatory power, as it can only directly explain small parts of existing landscapes, such as dissected fragments of former erosion surfaces.
- It is also speculative due to the significant modification of old erosion surfaces by subsequent processes, making it challenging to determine their original forms and heights accurately.
- Dating of erosion surfaces remains speculative due to the lack of valid geological evidence supporting precise dating methods.
(II) QUANTITATIVE AND EMPIRICAL APPROACH
- The quantitative or empirical approach serves as an alternative to the historical approach, especially suitable for studying large-scale geomorphological features where historical evidence is scarce due to extensive erosion and dissection.
- It involves measuring the geometric properties of landscape elements and interpreting them quantitatively to understand geomorphic processes.
- A fluvially originated drainage basin often serves as an ideal unit for morphometric studies, involving:
- Linear aspect: Determining hierarchical stream orders, computing stream numbers, bifurcation ratios, measuring stream lengths and basin areas, and establishing relationships between these variables.
- Areal aspect: Measuring basin shapes, computing stream frequency, drainage density, and drainage texture, and presenting spatial patterns cartographically.
- Relief aspect: Computing altimetric, hypsometric, and clinographic variables, determining relationships between area and height, height and slope angles, identifying erosion surfaces through altitudinal frequency maxima, calculating hypsometric and erosion integrals to understand erosion cycles, and measuring relative reliefs, dissection indices, ruggedness indices, and slope profiles.
- Developed in the U.S.A. in the 1940s, this approach has been widely adopted by geomorphologists worldwide for its systematic and quantitative analysis capabilities.
- However, results from morphometric analyses can sometimes be misleading or erroneous if not verified through field checks, potentially leading to incorrect conclusions about geomorphological processes.
(Ill) REGIONAL APPROACH
- Regional approach involves studying the landscape assemblage of a geomorphic region at large spatial and long temporal scales, encompassing mega-geomorphology and meso-geomorphology.
- It includes theoretical studies of the cyclic evolution of landforms and practical investigations of denudation chronology across spatial scales ranging from regional to continental.
- Approaches to mega-scale landform study can be categorized into three sub-categories:
- Explanation of present landscape characteristics and their evolution with reference to paleo-processes, spanning spatial scales from regional to sub-continental areas and temporal scales from millions to tens of thousands of years.
- Examination and explanation of present processes and the dynamic balance between process and form on sub-continental and regional scales, focusing on temporal scales from 1 to 100 years.
- Examination and explanation of significant determinants of geomorphological processes such as climatic changes, sea-level fluctuations, and regional to global tectonics.
- The goals of regional approach in mega-geomorphology include achieving an accurate understanding of past environmental conditions and associated processes, describing how and when these processes shaped the Earth’s surface, and developing models of the dynamic interaction between process and form.
- Analogous to the historical approach in landscape studies, the regional approach emphasizes understanding landscape evolution over long time spans and large spatial extents.
- However, in recent times, the historical or regional approach has been somewhat overshadowed by the process-form approach, which focuses on micro-geomorphology at small spatial and temporal scales.
(IV) SYSTEMATIC (FUNCTIONAL ) APPROACH
- Systematic approach in landform studies focuses on measuring and analyzing the operation of geomorphological processes that shape different landform suites under varying environmental conditions.
- The conceptual foundation of systematic approach involves functional studies of contemporary processes and the behavior of earth materials that can be directly observed, aiding in understanding both the maintenance and changes in landforms (Chorley, Schumm, and Sugden, 1985).
- Emphasis in the systematic approach is placed on observing and monitoring present-day processes at small spatial and short temporal scales, establishing causal relationships between processes and landform evolution, which forms the basis of process geomorphology.
- Process-form approach within systematic geomorphology emphasizes understanding erosional and depositional processes, their mechanics, rates of operation, and their role in explaining past evolution and predicting future changes.
- The systematic approach is further categorized based on major factors influencing landscape development, including process-form and structure-form approaches.
- Geomorphology’s independent existence as a discipline hinges on its ability to explain relationships between form and process, both historically and currently, and to elucidate how forms evolve over time (Rita Gardiner and Helen Scoging, 1983).
- Tasks of geomorphologists include detailed instrumentation and study of micro-processes to understand their physical and chemical impacts, interactions, and responses in shaping morphological features.
- Geomorphologists also reconstruct the chronology of environmental changes, identify paleo-processes, and explore their probable relationships with existing landforms.
- Analyzing dynamic systems at mega-scales (regional and continental) is crucial, as the controlling variables for landform development can vary significantly across different scales.
- Integrating these aspects enables a comprehensive understanding, modeling, and prediction of the morphological development of Earth’s surface.
C) RESEARCH METHODS
- R.J. Chorley (1966) outlines three essential steps and methods of data acquisition in geomorphology, which ultimately support theoretical work.
- Integrated approaches to research methods in geomorphology, according to Chorley, encompass field observations, laboratory observations, office observations, and theoretical work.
- Field observations play a significant role in geomorphological research, regardless of the specific study aim or methodological approach (C.A.M. King, 1966).
- Field observation methods include both qualitative and quantitative approaches, tailored to the objectives of landform studies.
- Geomorphologists practicing denudation chronology historically relied on qualitative field observations and subjective map analysis to understand landscape evolution and erosion surfaces at regional and mega scales.
- Functional and process-form approaches to landform studies emphasize the need for accurate quantitative data on landform morphology, processes, and materials (rocks, soils).
- Quantitative data acquisition in the field involves numerical measurements of landform characteristics such as slope angles, absolute and relative reliefs, and various dimensional properties of landform components.
- For processes like fluvial systems, field measurements include discharge, infiltration rates, evaporation rates, sediment load, rainfall, and runoff, facilitated by appropriate instruments.
- Laboratory observations in geomorphology encompass experimental studies and numerical measurements conducted on samples collected from the field.
- Examples of laboratory studies include analyzing the chemical and mechanical properties of soil and rock samples, determining chemical properties of water, and measuring grain sizes and chemical properties of suspended sediment loads.
- Controlled experiments in laboratories help measure phenomena like the nature and rate of rill and gully development, meander formation rates, soil erosion rates, sedimentation rates, and nick point recession rates.
- These methods are essential for understanding processes and landforms across various environmental conditions, ensuring robust data collection and empirical validation in geomorphological research.
- Office observation in geomorphology involves deriving data through map analysis, such as detailed map work. Today, extensive datasets are derived using various techniques from topographical maps, aerial photographs, and satellite images, focusing on different aspects of landforms.
- Geomorphologists utilize air photographs and satellite imagery to measure and derive data of geomorphological significance at regular intervals, enabling monitoring of geomorphic changes over time.
- Savindra Singh and Alok Dubey’s work (1991-1995) on gully erosion and management in India’s sub-humid tropical riverine alluvial environments exemplifies comprehensive geomorphological studies. They surveyed gully basins multiple times, tracing contours at one-meter intervals to derive morphometric data. They collected meteorological, hydrological, and geomorphological data through field instrumentation during wet monsoon months, processed mass datasets in computer labs, and analyzed water and sediment loads in geomorphological laboratories.
- The quantitative approach in geomorphology facilitated the development of morphometric analysis of fluvially originated drainage basins since the 1940s.
- Morphometric analysis includes:
- Linear aspects: hierarchical orders of streams, stream numbers, stream lengths, sinuosity, and meander properties derived from topographical maps.
- Areal aspects: stream frequency, drainage density, drainage texture, etc., also derived from topographical maps of varying scales.
- Relief aspects: relative relief, average slope, dissection index, altimetric, hypsometric, and clinographic properties.
- Theoretical work in geomorphology involves processing data and formulating models and theories, such as the laws of stream number and stream lengths (R.E. Horton), and the development of mathematical models to understand geomorphic processes and landform evolution.
D) METHODS OF ANALYSIS
- Inductive method in geomorphology involves arranging unordered facts in logical order based on correct definition and classification of observed phenomena.
- The process includes successive steps where data are collected first, defined, classified, and then lead to inductive generalization.
- Final conclusions and formulation of laws and theories are derived from the inductive process, providing satisfactory explanations for geomorphological problems.
- Inductive method is suited for simpler problems where wide observation and relevant data are available, minimizing the need for theoretical reasoning initially.
- Shortcomings of the inductive method include potential biases in data collection and the risk of overlooking geomorphologically significant facts that do not align with the final conclusion.
- There is a risk of deriving questionable or false conclusions if relevant data points are omitted or underrepresented in the analysis.
- Despite its drawbacks, providing an indication of the final conclusion early in the argument can sometimes aid in the clarity and direction of the research process.
- The deductive method in geomorphology starts with formulating a tentative hypothesis about the geomorphic problem being investigated.
- Consequences of the hypothesis are deduced in advance, guiding the collection of relevant facts and field observations.
- Actual observations are compared with deduced consequences to evaluate whether the hypothesis is supported or rejected.
- If the hypothesis is not successful, it is revised and the process is repeated; if successful, it may lead to the formulation of laws and theories explaining the real world.
- Weaknesses of the deductive method include potential bias in data collection towards facts that validate the hypothesis, while ignoring contradictory evidence.
- The quality of results depends on the accuracy of deductions and the validity of comparisons between deduced and observed facts.
- The analytical method involves formulating multiple alternative hypotheses and collecting data according to each hypothesis.
- By comparing observed facts and deductions from all alternative hypotheses, the analytical method seeks to identify the hypothesis that best fits the observations.
- This method aims to overcome biases inherent in both inductive and deductive methods by evaluating multiple hypotheses objectively.
- Accuracy, quantitative measurement, and systematic observation are essential in all methods of geomorphological analysis, alongside creativity and integrity in hypothesis development and testing.